top of page
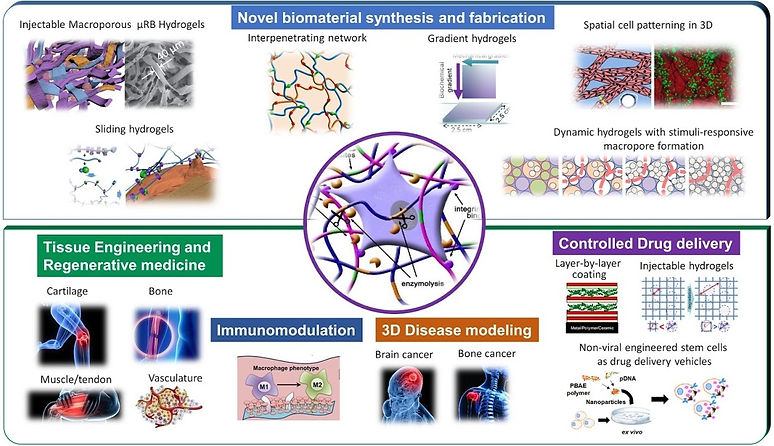
Microribbons | Sliding Hydrogels | Regenerative Medicine | 3D Disease Models | Biomaterials Fabrication | Drug Delivery | Immunomodulation
Microribbons
Microribbons
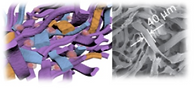
Conventional hydrogels are often nanoporous, lack macroporosity desirable for cell and tissue growth, and exhibit poor mechanical properties when subject to mechanical loading. Our patented µRB- based hydrogel technology was specifically designed to overcome these limitations by combines injectability with macroporosity, and exhibits “shock-absorbing” mechanical properties. You can think of these µRBs like legos: each piece can be tuned with tunable biochemical and mechanical properties, and they can be easily mixed and match through compatible intercrosslinking chemistry to promote desirable cell fates and tissue responses. We can also incorporate nanoparticles into the µRB scaffold to enable tunable in situ release of soluble factors.
Sliding Hydrogels
Sliding Hydrogels
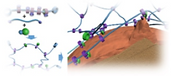
Depending on the crosslinking mechanisms, hydrogels can be broadly divided into two categories: physically-crosslinked hydrogels or covalently crosslinked hydrogels. Physical hydrogels are highly mobile but lack stability, and covalently crosslinked hydrogels are stable but do not offer any molecular mobility. How molecular mobility modulate cell fates in 3D remains unknown due to lack of biomaterials tools to interrogate such questions. To fill in these gaps of knowledge, our lab has recently reported sliding hydrogels as a 3D stem cell niche, which exhibits stability comparable to that of chemical hydrogels, yet allowing tunable molecular mobility due to its sliding/mobile crosslinks and biochemical ligands. The molecular mobility of this sliding hydrogel allows stem cells to reorganize the surrounding ligands in 3D, supporting more efficient stem cell differentiation toward various lineages.
Regenerative Medicine
Regenerative Medicine
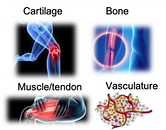
To translate our research from bench to bedside, a major effort of our research is to develop novel therapies to help regenerate tissues lost due to aging, diseases, trauma or cancer. In particular, we are interested in treating musculoskeletal diseases, cardiovascular diseases and cancer. Our strategy often involves customized designed 3D scaffolds with tunable niche cues (mechanical, biochemical, degradation, mobility, topographical cues etc), which can promote tissue regeneration through either directing stem cell differentiation or immunomodulation. We design 3D in vitro models to help determine the optimal scaffold design, and further validate their safety and efficacy using relevant animal disease models in vivo.
Immunomodulation
Immunomodulation
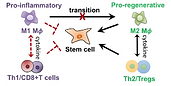
Immune cells are the first responders to tissue injury to initiate the inflammatory cascade and
secrete paracrine signals to recruit additional cell types for healing. Previous tissue engineering
research largely focused on targeting stem cells. Recent studies suggest biomaterials can also
modulate tissue regeneration via immunomodulation. However, previous studies were limited to
soft-tissue defects, and how tuning biomaterials compositions impact immune cell response in
critical-sized bone defect remains largely unknown. Our research seeks to harness
macroporous scaffolds with tunable compositions or surface coatings to enhance bone
regeneration in critical-sized defects through immunomodulation. We also seek to evaluate
the effect of ageing on biomaterials-mediated bone regeneration, and develop
immunomodulatory strategies to enhance bone healing in aged animal models.
3D Disease Models
3D Disease Models
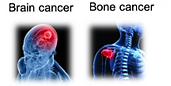
Current experimental models for cancer research primarily rely on 2D culture or mouse models. However, cancer cells grown in 2D culture generally fail to retain critical phenotypes of tumors in vivo, and are poor at predicting drug responses in vivo. Mouse models are more physiologically relevant, but are costly and impractical for high-throughput drug screening. Leveraging on our strong expertise in hydrogel design, we engineer 3D cancer models to better recapitulate in vivo tumor invasion, signaling and drug responses than 2D cultures. Such 3D in vitro disease models holds great potential for mechanistic studies and for drug screening and discovery.
Biomaterials Fabrication
Novel Biomaterials Fabrication

In addition to the microribbon and sliding hydrogel platforms, our lab has also developed various biomaterials with unique structures to achieve specific design needs or tissue functions such as: (1) allow decoupling niche cues independently (Interpenetrating network hydrogels), (2) introducing gradient niche cues to mimic zonal organization (2D or 3D gradient hydrogels), (3) dynamic hydrogels with stimuli-responsive macropore formation, (4) spatially pattern microchannels inside 3D hydrogels.
Drug Delivery
Drug Delivery
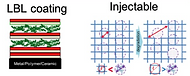
Ex vivo culture is costly and substantially increase the barrier for regulatory approval and clinical translation. In situ drug delivery systems would be highly desirable. Towards this goal, our group has developed various in situ drug delivery platforms: including layer-by-layer coating,
injectable hydrogels or implantable macroporous solid scaffolds to promote tissue formation
in vivo.
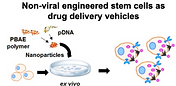
Program stem cells as drug-delivery vehicles
Many disease processes could be treated much more effectively if we could deliver the right therapeutics to the right place at the right time. However, effective targeting and delivery remains challenging. Our strategy leverages the “inherent GPS” of stem cells for effective targeting to disease sites and pursues a safer, non-viral gene-delivery method to overexpress therapeutic factors in situ, to either promote tissue regeneration, or to eradicate infiltrating cancer satellite cells.
Microribbon Publications
Microribbons
Han LH, Yu S, Wang TY, Behn AW, Yang F*. “Microribbon-like Elastomers for Fabricating Macroporous and Highly Flexible Scaffolds that Support Cell Proliferation in 3D”, Advanced Functional Materials, 2013 Jan; 23: 346–358. DOI: 10.1002/adfm.201201212 | Article
Han LH, Tong X, Yang F*. “Photocrosslinkable PEG-based Microribbons for Forming 3D Macroporous Scaffolds with Decoupled Niche Properties”. Advanced Materials Epub 2013 Dec 17, DOI:10.1002/adma.201304805 | Article
Gegg C, Tong X, Yang F*. “Mixed composition microribbon hydrogels induce rapid and synergistic cartilage regeneration by MSCs in 3D via paracrine signal exchange”. ACS Biomaterials Science & Engineering, 2020 May, DOI: 10.1021/acsbiomaterials.0c00131 | Article
Tang Y, Tong X, Conrad B, Yang F*. "Injectable and in situ Crosslinkable Gelatin Microribbon Hydrogels for Stem Cell Delivery and Bone Regeneration in vivo". Theranostics, 2020 May; 10(13): 6035–6047. DOI: 10.7150/thno.41096, PMID: 32483436. | Article
Conrad B, Hayashi C, Yang F* . “Gelatin-based microribbon hydrogels support robust MSC osteogenesis across a broad range of stiffness”, ACS Biomaterials Science & Engineering, 2020 Apr, 6(6): 3454-3463; | Article
Barati D, Watkins K, Wang Z, Yang F* . “Injectable and Crosslinkable PLGA‐Based Microribbons as 3D Macroporous Stem Cell Niche”. Small, 2020 Jun; 16(22):e1905820. DOI: 10.1002/smll.201905820. PMID: 32338432 | Article
Barati D*, Gegg C*, Yang F*. "Nanoparticle-mediated TGF-β Release from Microribbon-based Hydrogels Accelerates Stem Cell-based Cartilage Formation in vivo." Annals of Biomedical Engineering 2020 Jul; 48 (7): 1971-1981. DOI: 10.1007/s10439-020-02522-z. Epub 2020 May 6. PMID: 32377980. | Article
Slidin Gel Publicatons
Sliding Hydrogels
Tong X, Yang F*. "Sliding hydrogels with mobile molecular ligands and crosslinks as 3D stem cell niche" Advanced Materials, 2016 Sep;28(33):7257-63. DOI: 10.1002/adma.201601484. Epub 2016 Jun 15. | Article
Regenerative Medicine Publications
Regenerative Medicine
Ueno M, Zhang N, Hirata H, Barati D, Utsunomiya T, Shen H, Lin T, Maruyama M, Huang E, Yao Z, Wu JY, Zwingenberger S, Yang F, Goodman SB. Sex Differences in Mesenchymal Stem Cell Therapy With Gelatin-Based Microribbon Hydrogels in a Murine Long Bone Critical-Size Defect Model. Front Bioeng Biotechnol. 2021 Oct 19;9:755964. doi: 10.3389/fbioe.2021.755964. PMID: 34738008 | Article
Tang Y, Tong X, Conrad B, Yang F*. "Injectable and in situ-Crosslinkable Gelatin Microribbon Hydrogels for Stem Cell Delivery and Bone Regeneration in vivo." Theranostics, 2020 May; 10(13):6035–6047. DOI: 10.7150/thno.41096, PMID: 32483436. | Article
Murphy M, Koepke LS, Lopez MT, Tong X, Ambrosi TH, Gulati GS, Marecic O, Wang Y, Ransom RC, Hoover MY, Steininger H, Zhao LM, Walkiewicz MP, Quarto N, Levi B, Wan D, Weissman IL, Goodman SB, Yang F*, Longer MT, Chan CK. "Articular cartilage regeneration by activated skeletal stem cells." Nature Medicine, 2020 Aug 17, DOI: 10.1038/s41591-020-1013-2. PMID: 32807933. | Article
Han LH, Chung MT, Deveza L, Jiang X, Conrad B, Wang A, Butte MJ, Longaker MT, Wan D, Yang F*. “Microribbon-based hydrogels accelerate stem cell-based bone regeneration in a mouse critical-size cranial defect model". J Biomed Mater Res A. 2016 Jun;104(6):1321-31. | Article
Conrad B, Han L, Yang F*. "Gelatin-based microribbon hydrogels accelerate cartilage formation by mesenchymal stem cells in 3D", Tissue Engineering Part A , 2018 Jun 21. | Article
Stanton AE, Tong X, Jing S, Behn AW, Storaci H, Yang F. Aligned microribbon scaffolds with hydroxyapatite gradient for engineering bone-tendon interface. Tissue Eng Part A, 2022 Aug;28(15-16):712-723. doi: 10.1089/ten.TEA.2021.0099. PMID: 35229651 https://pubmed.ncbi.nlm.nih.gov/35229651/ | Article
Lee S, Tong X, Han LH, Behn A, Yang F*. "Aligned microribbon-like hydrogels for guiding three-dimensional smooth muscle regeneration" Journal of Biomedical Materials Research: Part A. 2016 May;104(5):1064-71. | Article
Tevlin R, Seo E, Marecic O, McArdle A, Tong X, Zimdahl B, Malkovskiy A, Sinha R, Gulati G, Li X, Wearda T, Morganti R, Lopez M, Ransom RC, Duldulao CR, Rodrigues M., Nguyen A, Januszyk M, Maan Z, Paik K, Yapa KS, Rajadas J, Wan DC, Gurtner GC, Snyder M, Beachy PA, Yang F* , Goodman SB, Weissman IL, Chan C, Longaker MT. "Pharmacological rescue of diabetic skeletal stem cell niches". Science Translational Medicine, 2017 Jan 11;9(372). pii: eaag2809. DOI: 10.1126/scitranslmed.aag2809. PMID:28077677. | Article
Immunomodulation
Su N, Villicana C, Barati D, Freeman P, Luo Y, Yang F. Stem Cell Membrane‐coated Microribbon Scaffolds Induce Regenerative Innate and Adaptive Immune Responses in a Critical‐Size Cranial Bone Defect Model. Advanced Materials. 2022 Dec:2208781. | Article
Ambrosi TH, Marecic O, McArdle A, Sinha R, Gulati GS, Tong X, Wang Y, Steininger HM, Hoover MY, Koepke LS, Murphy MP, Sokol J, Seo EY, Tevlin R, Lopez M, Brewer RE, Mascharak S, Lu L, Ajanaku O, Conley SD, Seita J, Morri M, Neff NF, Sahoo D, Yang F, Weissman IL, Longaker MT, Chan CKF. Aged skeletal stem cells generate an inflammatory degenerative niche. Nature. 2021 Sep;597(7875):256-262. doi: 10.1038/s41586-021-03795-7. PMID: 34381212 | Article
Hirata H, Zhang N, Ueno M, Barati D, Kushioka J, Shen H, Tsubosaka M, Toya M, Lin T, Huang E, Yao Z, Wu JY, Zwingenberger S, Yang F, Goodman SB. Ageing attenuates bone healing by mesenchymal stem cells in a microribbon hydrogel with a murine long bone critical-size defect model. Immun Ageing. 2022 Mar 12;19(1):14. | Article
Immunomodulation Publictions
3D Disease Modeling Publications
3D Disease Modeling
Sinha S, Ayushman M, Tong X, Yang F. Dynamically crosslinked poly(ethylene-glycol) hydrogels reveal a critical role of viscoelasticity in modulating glioblastoma fates and drug responses in 3D. Adv Healthc Mater. 2023 Jan; 12(1):e2202147. doi: 10.1002/adhm.202202147. Epub 2022 Nov 16. https://pubmed.ncbi.nlm.nih.gov/36239185/ | Article
González Díaz EC, Lee AG, Sayles LC, Feria C, Sweet-Cordero EA, Yang F. A 3D Osteosarcoma Model with Bone-Mimicking Cues Reveals a Critical Role of Bone Mineral and Informs Drug Discovery. Adv Healthc Mater. 2022 Jun 29:e2200768. doi: 10.1002/adhm.202200768. PMID: 35767377 https://onlinelibrary.wiley.com/doi/10.1002/adhm.202200768 | Article
González Díaz EC, Sinha S, Avedian RS, Yang F*. “Tissue-engineered 3D models for elucidating primary and metastatic bone cancer progression” Acta Biomater. 2019 Nov;99:18-32. PMID: 31419564. | Article
Wang C, Sinha S, Jiang X, Murphy L, Fitch S, Wilson C, Grant G, Yang F*. “Matrix stiffness modulates patient-derived glioblastoma cell fates in 3D hydrogels”, Tissue Engineering Part A,2020 July, DOI: 10.1089/ten.TEA.2020.0110. PMID: 32731804. | Article
Zhu D, Trinh P, Li J, Grant GA, Yang F*. “Gradient hydrogels for screening stiffness effects on patient-derived glioblastoma xenograft cell fates in 3D”, J Biomed Mater Res A, 2020 Aug 30. DOI: 10.1002/jbm.a.37093. PMID: 32862485. Online ahead of print. | Article
Wang C, Sinha S, Jiang X, Fitch S, Wilson C, Caretti V, Ponnuswami A, Monje M, Grant G, Yang F*. “A comparable study of brain tumor cells from different age and anatomical locations using 3D biomimetic hydrogels”, Acta Biomater, 2020 Sep 7;S1742-7061(20)30521-3. DOI: 10.1016/j.actbio.2020.09.007. PMID: 32911104. | Article
Wang C, Tong X, Jiang X, Yang F*. “Effect of matrix metalloproteinase-mediated matrix degradation on glioblastoma cell behavior in 3D PEG-based hydrogels”. J Biomed Mater Res A. 2017;105(3):770-8. Epub 2016/10/23. | Article
Wang C, Tong X, Yang F*. “Bioengineered 3D Brain Tumor Model To Elucidate the Effects of Matrix Stiffness on Glioblastoma Cell Behavior Using PEG-Based Hydrogels”. Molecular Pharmaceutics. 2014;11(7):2115-25. | Article
Novel Biomaterials Publications
Novel Biomaterials Fabrication
Tong X, Yang F*. "Engineering Interpenetrating Network Hydrogel as Biomimetic Cell Niche with Independently Tunable Biochemical and Mechanical Properties." Biomaterials 2014 Feb;35(6):1807-15. 2. | Article
Zhu D, Tong X, Trinh P, Yang F*."Mimicking cartilage tissue zonal organization by engineering tissue-scale gradient hydrogels as 3D cell niche". Tissue Eng Part A. 2018 Jan;24(1-2):1-10. | Article
Zhu D,Trinh P, Liu E, Yang F*."Biochemical and Mechanical Gradients Synergize to Enhance Cartilage Zonal Organization in 3D". ACS Biomaterials Science & Engineering. August 2018, | Article
Han LH, Lai J, Yu S, Yang F*. "Dynamic Tissue Engineering Scaffolds with Stimuli-Responsive Macroporosity Formation." Biomaterials, 2013, 34(17): 4251-8. 5. | Article
Madl C, Keeney M, Li X, Han LH, Yang F*. "Co-release of cells and polymeric nanoparticles from sacrificial microfibers enhances non-viral gene delivery inside 3D hydrogels." Tissue Engineering Part C Methods, 2014 Oct;20(10):798-805. DOI: 10.1089/ten.TEC.2013.0669. Epub 2014 Apr 4. | Article
Drug Delivery Publications
Drug Delivery
Keeney M, Waters H, Barcay K, Jiang XY, Yao Z, Pajarinen J, Egashira K, Goodman S, Yang F*. "Mutant MCP-1 Protein Delivery from Layer-by-Layer Coatings on Orthopaedic Implants to Modulate Inflammatory Response". Biomaterials. 2013 Dec; 34(38): 10287-95. | Article
Keeney M, Onyiah S, Zhang Z, Tong X, Han LH, Yang F*. "Modulating Polymer Chemistry to Enhance Non-viral Gene Delivery inside Hydrogels with Tunable Matrix Stiffness". Biomaterials. 2013 Dec;34(37):9657-65. | Article
Keeney M*, Ong SG*, Padilla A, Yao Z, Goodman S, Wu J, Yang F*. "Development of Poly (beta-amino esters)-Based Biodegradable Nanoparticles for Non-viral Delivery of Minicircle DNA." ACS Nano., 2013, 7 (8), pp 7241-7250. (*: equal contribution). | Article
Jiang X, Fitch S, Wang C, Wilson C, Li JF, Song B, Grant G, Yang F*. "Nanoparticle engineered TRAIL-overexpressing stem cells target and eradicate patient-derived glioblastoma xenograft in vivo". Proc Natl Acad Sci USA, 2016 Nov 29;113(48):13857-13862. Epub 2016 Nov 14. | Article
Jiang X, Wang C, Fitch S, Yang F*."Targeting tumor hypoxia using nanoparticle-engineered CXCR4-overexpressing adipose-derived stem cells" Theranostics. 2018 Feb 2;8(5):1350-1360. | Article
Deveza L, Choi J, Lee J, Huang N, Cooke J, Yang F*. "Polymer-DNA nanoparticle-induced CXCR4 overexpression improves stem cell engraftment and tissue regeneration in a mouse hindlimb ischemia model. "Theranostics, 2016 May 23;6(8):1176-89. DOI: 10.7150/thno.12866. eCollection 2016. | Article
bottom of page